Biocatalysis: Case Studies
In this lesson, we will look at some examples where biocatalysis has been employed for the synthesis of Active Pharmaceutical Ingredients (APIs). This will demonstrate the potential impact of biocatalysis in the pharmaceutical industry, in terms of a move towards lower environmental impact manufacturing processes, as well as making important medicines on the required scale at an affordable price.
CHEM21 Case Study: Reductive Amination
Chiral amines are an important class of organic compounds that serve as key intermediates in the synthesis of a variety of biologically active molecules.[1] The formation of C=N bonds by the condensation of carbonyls and amines, followed by reduction of the in situ formed imine represents the most straightforward strategy for the generation of chiral amines.
Most work has focused on the transition metal-catalyzed asymmetric hydrogenation of imines and particular attention was paid to the reductive amination of carbonyl compounds with primary amines. Several reagents and combinations have been reported through the years with a predominant role being played by modified borohydride reagents.[2] Inspired by the biological system NAD(P)H, Hantzsch ester has been employed as a hydrogen donor in combination with chiral phosphoric acid for the enantioselective reductive amination of aliphatic ketones to furnish amines with excellent enantioselectivities.[3][4][5]
The application of biocatalysis is an important complement to chemical catalysis for chiral amine synthesis in water. Two main types of enzymes were investigated so far for the transformation of carbonyl groups into amines: amino acid dehydrogenases and w-transaminases. Researchers within CHEM21 have identified imine reductases [6] as biocatalyst for chemo- and stereoselective intermolecular reductive aminations. This novel imine reductase-catalyzed approach constitutes a direct method for the synthesis of valuable amines under mild reaction conditions.[7]
![Scheme 1: Classical and novel reductive amination (Hauer et al, 2015; Chusov and List, 2014 [7][8])](/wp-content/themes/yootheme/cache/45/94f1b722b6c392b844e749765d9a40f8-45772be9.jpeg)
This approach represents a simple and complementary strategy for the generation of novel carbon nitrogen bonds in aqueous reaction media. These reactions proceed efficiently for a variety of carbonyl compounds and amine nucleophiles. Low amounts of the imine intermediate led to the formation of valuable amines in good yields and high selectivities. A highly chemo- and stereoselective imine reductase was applied in reductive aminations of a panel of carbonyls and amine nucleophiles to afford primary and secondary amine products in moderate to high yield as well as enantiomeric excess.
CHEM21 Case Study: Whole-cell Imine Reductases for Asymmetric Reduction of Cyclic Amines
As important structural motifs, chiral amines are found in a variety of industrially relevant compounds such as pharmaceuticals, agrochemicals and fine chemicals.[9] A variety of methods are available for their synthesis that include metal catalysed and organocatalysed asymmetric synthesis; despite this, the most commonly used method in industry to obtain chiral amines is through recrystallization (Scheme 1) or kinetic resolution,[10][11] with chiral cyclic amines more commonly obtained through recrystallisation. As such, the maximum theoretical yield for the desired enantiomer is capped at 50%. The use of co-crystallising agent also means that the process uses up a stoichiometric amount of reagent that is destined for waste, essentially making the process mass intensive.

The alternative is to consider the use of biocatalysis for the synthesis of chiral cyclic amines, in particular those biocatalysts that can be modified and enhanced through protein engineering and directed evolution. These include monoamine oxidases (MAO-N), phenyl alanine ammonia lyases and aminotransferases; however, the majority of these biocatalysts are limited to the synthesis of primary amines. On the other hand, the asymmetric reduction of imines offers an alternative route for the generation of these molecules; this approach has been examined in chemocatalytic approaches as well as in tandem with biocatalysts, however studies into the enzymatic approach have been limited.[12]
Imine reductases (IREDs) catalyse the reduction of imines to amines by using NADH or NADPH as cofactors. These enzymes from different strains of Streptomyces sp. have been previously shown to reduce prochiral cyclic imines to their corresponding chiral amines, and the sequences for the two enantiocomplimentary IREDs from Streptomyces sp. GF3587 and Streptomyces sp. GF3546 have been reported.[13] Researchers in the CHEM21 consortium have investigated the applicability and scope in substrate of both R– and S-imine reductases by expressing them in Escherichia coli. and found that the (S)-IRED can mediate the enantioselective reduction of five-, six- and seven-membered imines as well as dihydroquinolines, β-carbolines and iminium ions in high enantiomeric excess (ee).[14] The (R)‑IRED, overexpressed in E. coli also displayed a broad substrate scope, allowing the highly enantioselective preparation of chiral cyclic secondary amines that carry broad structural features, moreover the enzyme showed higher activity than has been previously reported, demonstrating its potential for applicability on industrial scale (Scheme 2).[12]
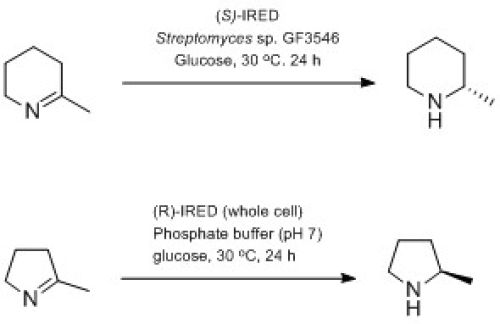
CHEM21 Case Study: Biocatalysis in Bio-derived Solvents
This case study was provided by Giulia Paggiola during her time at the Green Chemistry Centre of Excellence, University of York
One of the major obstacles to industrial uptake of biocatalysis is the difficulty in achieving similar reaction conditions to those of organic chemical reactions.[15] In biotransformations, water is traditionally used as the reaction solvent. However, there are many associated issues with the use of water as the reaction medium. These include issues with the solubility of the organic substrates (which generally require the addition of an organic water-soluble co-solvent), purification and enzyme recovery, as well as the incurred cost and environmental impact of contaminated aqueous waste treatment.[16]
These issues can be overcome by the use of organic solvents as the reaction media, an approach that is well established in academic research but is not common in industrial applications.[17][18] However, the approach to the use of organic solvents in enzymatic reactions has been somewhat incidental. There have been limited studies into the use of “green” solvents as reaction media for enzymatic transformations and include the use of ionic liquids, supercritical CO2 and some bio-derived solvent.[19][20] The CHEM21 researchers investigated a more systematic approach in assessing the behaviour of supported Candida Antarctica lipase B (Novozyme 435) in 24 organic solvents including classical as well as bio-derived solvents (Figure 1); this was investigated through carrying out a kinetic study of the reaction of hexanol with dodecanoic acid to produce the industrially relevant hexyl laurate, used in the personal care and cosmetics industries.[16]
![Figure 1: Kinetic Screening for Novozyme 435 mediated esterification reaction of hexanol with dodecanoic acid, in a variety of organic solvents (Reproduced from G. Paggiola et al [2] with permission of The Royal Society of Chemistry)](https://learning.acsgcipr.org/wp-content/uploads/2022/07/201e7651800ee0c3391e7f2556b08b15-300x273.png)
The researchers observed a correlation between high activity of Novozyme 435 and solvent properties, specifically hydrogen-bond accepting ability and molar volume. In comparison to previous studies, the study found that molar concentration was an important factor; it is hypothesised that this is related to the partition coefficient (log P) and its ability to remove structural water from the enzyme active site. The bioderived solvents that performed best were limonene and p-cymene with the former outperforming the classically used hexane, offering an effective and sustainable approach for industrial fatty acid ester synthesis.[16]
API case studies
- D. Ghislieri and N. J. Turner, Biocatalytic Approaches to the Synthesis of Enantiomerically Pure Chiral Amines, Top Catal, 2014, 57, 284-300.
- T. C. Nugent and M. El-Shazly, Chiral Amine Synthesis – Recent Developments and Trends for Enamide Reduction, Reductive Amination, and Imine Reduction, Advanced Synthesis & Catalysis, 2010, 352, 753-819.
- V. N. Wakchaure, J. Zhou, S. Hoffmann and B. List, Catalytic Asymmetric Reductive Amination of α-Branched Ketones, Angewandte Chemie International Edition, 2010, 49, 4612-4614.
- W. Li and X. Zhang, Formation of Amines, Springer, Berlin, 2014.
- S. Hoffmann, A. Majeed Seayad and B. List, A Powerful Brønsted Acid Catalyst for the Organocatalytic Asymmetric Transfer Hydrogenation of Imines, Angewandte Chemie International Edition, 2005, 44, 7424-7427.
- P. N. Scheller, S. Fademrecht, S. Hofelzer, J. Pleiss, N. J. Leipold Friedemann and Turner, B. M. Nestl and B. Hauer, Enzyme toolbox: novel enantiocomplementary imine reductases, Chembiochem, 2014, 15, 2201–2204.
- P. N. Scheller, M. Lenz, B. Hammer Stephan C and Hauer and B. M. Nestl, Imine Reductase-Catalyzed Intermolecular Reductive Amination of Aldehydes and Ketones, ChemCatChem, 2015, 7, 3239–3242.
- D. Chusov and B. List, Reductive Amination without an External Hydrogen Source, Angewandte Chemie International Edition, 2014, 53, 5199-5201.
- M. E. Welsch, S. A. Snyder and B. R. Stockwell, Privileged scaffolds for library design and drug discovery, Current Opinion in Chemical Biology, 2010, 14, 347-361.
- D. L. Silverio, S. Torker, T. Pilyugina, E. M. Vieira, M. L. Snapper, F. Haeffner and A. H. Hoveyda, Simple organic molecules as catalysts for enantioselective synthesis of amines and alcohols, Nature, 2013, 494, 216-221.
- M. Binanzer, S. – Y. Hsieh and J. W. Bode, Catalytic Kinetic Resolution of Cyclic Secondary Amines, Journal of the American Chemical Society, 2011, 133, 19698-19701.
- S. Hussain, F. Leipold, E. Man Henry and Wells, S. P. France, G. Mulholland Keith R and Grogan and N. J. Turner, An (R)-Imine Reductase Biocatalyst for the Asymmetric Reduction of Cyclic Imines, ChemCatChem, 2015, 7, 579–583.
- Patent EP2330190, 2011.
- F. Leipold, S. Hussain, D. Ghislieri and N. J. Turner, Asymmetric Reduction of Cyclic Imines Catalyzed by a Whole-Cell Biocatalyst Containing an (S)-Imine Reductase, ChemCatChem, 2013, 5, 3505-3508.
- S. Wenda, S. Illner, A. Mell and U. Kragl, Industrial biotechnology-the future of green chemistry?, Green Chem., 2011, 13, 3007-3047.
- G. Paggiola, A. J. Hunt, J. McElroy Con R and Sherwood and J. H. Clark, Biocatalysis in bio-derived solvents: an improved approach for medium optimisation, Green Chem., 2014, 16, 2107–2110.
- Enzyme Catalysis in Organic Synthesis, (ed. H. Gröger and O. May) Wiley-VCH Verlag GmbH & Co. KGaA, 2012.
- C. Jimenez-Gonzalez, P. Poechlauer, Q. B. Broxterman, B. – S. Yang, Dam Ende, J. Baird, C. Bertsch, R. E. Hannah, P. Dell’Orco, H. Noorman, S. Yee, R. Reintjens, A. Wells, V. Massonneau and J. Manley, Key Green Engineering Research Areas for Sustainable Manufacturing: A Perspective from Pharmaceutical and Fine Chemicals Manufacturers, Org. Process Res. Dev., 2011, 15, 900-911.
- M. J. Hernáiz, A. R. Alcántara, J. I. García and J. V. Sinisterra, Applied Biotransformations in Green Solvents, Chem. Eur. J., 2010, 16, 9422-9437.
- Z. Yang and W. Pan, Ionic liquids: Green solvents for nonaqueous biocatalysis, Enzyme Microb. Technol., 2005, 37, 19-28.