Specific Examples of the Fate of APIs
Listed below are 10 typical pharmaceutical molecules and their breakdown products under conditions likely to be encountered in the environment. A number of treatments collectively called advanced oxidation technologies/processes (AOT/AOP) can be used to treat waste water containing chemicals known to be recalcitrant and/or toxic in biological treatment/sewage treatment plants before discharge into the environment. Decomposition products from AOT/AOP processing have not been included since these technologies operate under harsh oxidative conditions that are never encountered in the environment. APIs may come into contact with milder technologies used to disinfect drinking water, which would lead to partial oxidation.
Whatever the mode of reaction, unless a molecule is mineralised, it should not be assumed that one or two transformations will render a toxic/recalcitrant molecule less so. Transformation may result in products with greater environmental issues than the original molecule.[1][2] Likewise, quantitifying the eco-toxicity of breakdown products computationally can present issues, e.g. with quantitative structure–activity relationship (QSAR) modelling since:
- It is often inaccurate for the parent pharmaceutical molecules;
- The assigned structures for the transformation products could be incorrect;
- An unambiguous answer can only be reached from physical testing.
The ten molecules have been selected to give examples of APIs across different therapeutic areas and to show examples of biotic, direct and indirect photolytic decomposition. Mainly, primary breakdown products are shown. These could be subject to further degradation processes.
1) Diclofenac
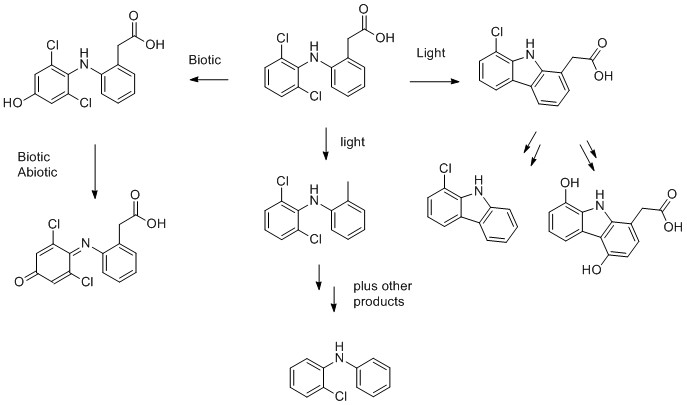
2) Fluoxetine
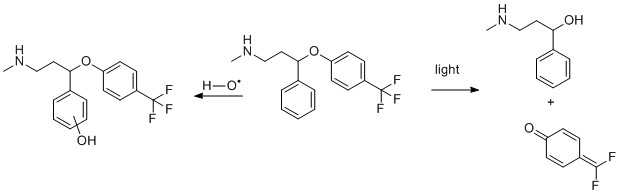
3) Paroxetine
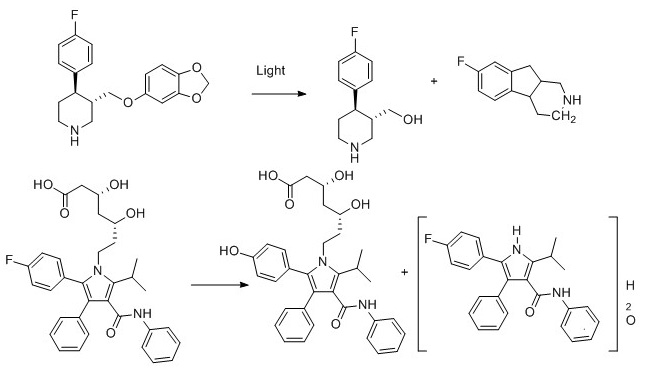
4) Atorvastin
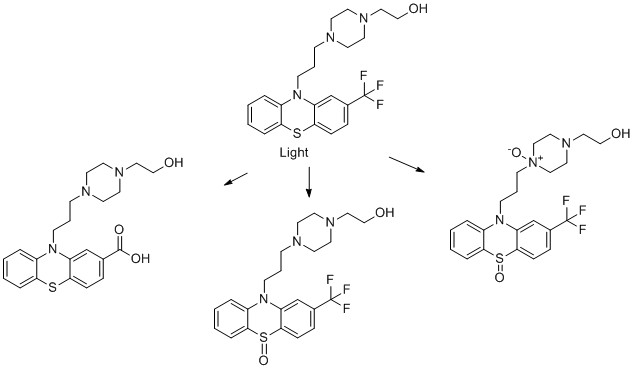
5) Fluphenazine
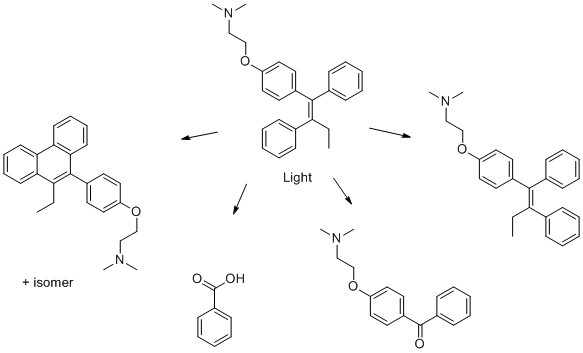
6) Tamoxifen
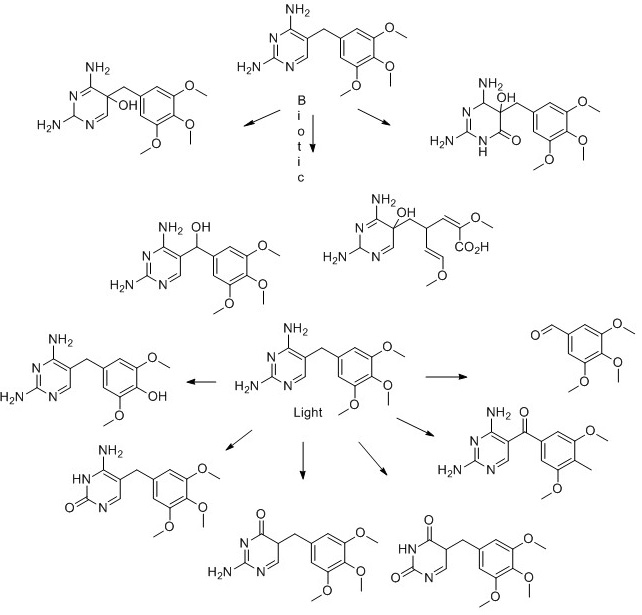
7) Trimethoprim
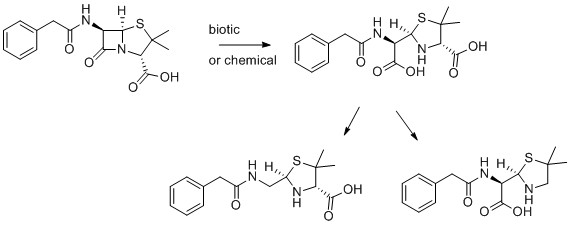
8) Penicillin G
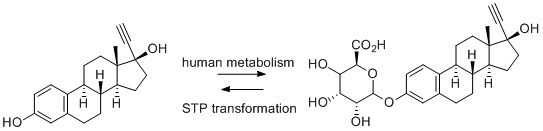
9) Ciproflaxacin
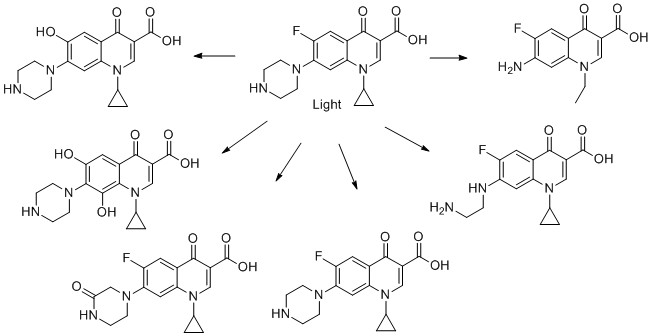
10) Valsartan
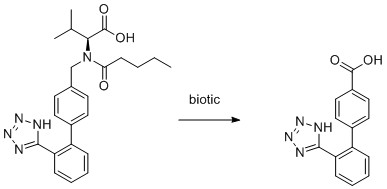
- M. Bergheim, R. Gminski, B. Spangenberg, M. Dębiak, A. Bürkle, V. Mersch-Sundermann, K. Kümmerer and R. Gieré, Recalcitrant pharmaceuticals in the aquatic environment: a comparative screening study of their occurrence, formation of phototransformation products and their in vitro toxicity, Environmental Chemistry, 2014, 11, 431-444.
- X. – H. Wang and A. Yu- Chen Lin, Is the phototransformation of pharmaceuticals a natural purification process that decreases ecological and human health risks?, Environmental Pollution, 2014, 186, 203-215.
- J. Gröning, C. Held, C. Garten, U. Claußnitzer, S. R. Kaschabek and M. Schlömann, Transformation of diclofenac by the indigenous microflora of river sediments and identification of a major intermediate, Chemosphere, 2007, 69, 509-516.
- D. E. Moore, S. Roberts-Thomson, D. Zhen and C. C. Duke, PHOTOCHEMICAL STUDIES ON THE ANTIINFLAMMATORY DRUG DICLOFENAC, Photochemistry and Photobiology, 1990, 52, 685-690.
- A. Agüera, L. A. Pérez Estrada, I. Ferrer, E. M. Thurman, S. Malato and A. R. Fernández-Alba, Application of time-of-flight mass spectrometry to the analysis of phototransformation products of diclofenac in water under natural sunlight, Journal of Mass Spectrometry, 2005, 40, 908-915.
- T. Poiger, H. – R. Buser and M. D. Müller, Photodegradation of the pharmaceutical drug diclofenac in a lake: Pathway, field measurements, and mathematical modeling, Environmental Toxicology and Chemistry, 2001, 20, 256-263.
- M. W. Lam, C. J. Young and S. A. Mabury, Aqueous Photochemical Reaction Kinetics and Transformations of Fluoxetine, Environmental Science & Technology, 2005, 39, 513-522.
- J. – W. Kwon and K. L. Armbrust, Hydrolysis and photolysis of paroxetine, a selective serotonin reuptake inhibitor, in aqueous solutions, Environmental Toxicology and Chemistry, 2004, 23, 1394-1399.
- M. W. Lam and S. A. Mabury, Photodegradation of the pharmaceuticals atorvastatin, carbamazepine, levofloxacin, and sulfamethoxazole in natural waters, Aquat. Sci., 2005, 67, 177-188.
- C. Trautwein and K. Kümmerer, Ready biodegradability of trifluoromethylated phenothiazine drugs, structural elucidation of their aquatic transformation products, and identification of environmental risks studied by LC-MS n and QSAR, Environ Sci Pollut Res, 2012, 19, 3162-3177.
- M. DellaGreca, M. Rosaria Iesce, M. Isidori, A. Nardelli, L. Previtera and M. Rubino, Phototransformation products of tamoxifen by sunlight in water. Toxicity of the drug and its derivatives on aquatic organisms, Chemosphere, 2007, 67, 1933-1939.
- J. J. Bergh, J. C. Breytenbach and P. L. Wessels, Degradation of trimethoprim, Journal of Pharmaceutical Sciences, 1989, 78, 348-350.
- P. Eichhorn, L. P. Ferguson, S. Pérez and D. S. Aga, Application of Ion Trap-MS with H/D Exchange and QqTOF-MS in the Identification of Microbial Degradates of Trimethoprim in Nitrifying Activated Sludge, Analytical Chemistry, 2005, 77, 4176-4184.
- C. Sirtori, A. Agüera, W. Gernjak and S. Malato, Effect of water-matrix composition on Trimethoprim solar photodegradation kinetics and pathways, Water Research, 2010, 44, 2735-2744.
- T. Yi, W. Barr and W. F. Harper, Electron density-based transformation of trimethoprim during biological wastewater treatment, Water Science and Technology, 2012, 65, 689-696.
- M. Bergheim, T. Helland, R. Kallenborn and K. Kümmerer, Benzyl-penicillin (Penicillin G) transformation in aqueous solution at low temperature under controlled laboratory conditions, Chemosphere, 2010, 81, 1477-1485.
- T. Haddad and K. Kümmerer, Characterization of photo-transformation products of the antibiotic drug Ciprofloxacin with liquid chromatography–tandem mass spectrometry in combination with accurate mass determination using an LTQ-Orbitrap, Chemosphere, 2014, 115, 40-46.
- J. Burhenne, M. Ludwig and M. Spiteller, Photolytic degradation of fluoroquinolone carboxylic acids in aqueous solution, Environ Sci Pollut Res, 1997, 4, 61-67.
- K. Nödler, O. Hillebrand, K. Idzik, M. Strathmann, F. Schiperski, J. Zirlewagen and T. Licha, Occurrence and fate of the angiotensin II receptor antagonist transformation product valsartan acid in the water cycle – A comparative study with selected β-blockers and the persistent anthropogenic wastewater indicators carbamazepine and acesulfame, Water Research, 2013, 47, 6650-6659.
- S. Kern, R. Baumgartner, D. E. Helbling, J. Hollender, H. Singer, M. J. Loos, R. P. Schwarzenbach and K. Fenner, A tiered procedure for assessing the formation of biotransformation products of pharmaceuticals and biocides during activated sludge treatment, Journal of Environmental Monitoring, 2010, 12, 2100-2111.
- Examining the Life Cycle
- Drivers Towards Whole-process Thinking
- Challenges in Effecting Change
- LCA Examples
- Primary Manufacturing
- Secondary Manufacturing
- Packaging
- Pharmaceuticals in the Environment (PIE)
- Appendix: Carbon Footprinting Assumptions
- Examining the Life Cycle: Quiz
- Examining the Life Cycle: Summary and Further Reading
- The Fate of APIs
- Benign by Design
- Life Cycle Impacts: Summary and Further Reading