Adsorption and Decomposition Pathways
Many APIs and their metabolites once excreted from the patient or discharged into water courses are recalcitrant to further breakdown in sewage treatment plants (STPs) and natural water courses (rivers, lakes etc.). When entering the environment via the patient, APIs may be excreted unchanged, as mixtures of unchanged API alongside one or several major metabolite(s), or completely metabolised to single or mixtures of metabolite(s).
In the environment, there may be no, partial or full chemical breakdown of the excreted compounds. As with metabolites, breakdown products can be more problematic than the parent compound – potentially more toxic and more recalcitrant.[1][2] Many APIs are not removed during typical sewage treatments, but can be adsorbed onto sewage sludge.
Therefore once released into the environment, an API or metabolite may:
- Decompose to innocuous fragments and eventually be mineralised – converted to carbon dioxide, nitrates, sulphates etc.
- Decompose to more recalcitrant fragments that are persistent.
- Be recalcitrant – i.e. does not decompose, and may or may not show undesirable biological activity.
- Bind to organic solids such as soils, river/lake sediments and sludge in STPs where it could accumulate or slowly decompose. This binding rapidly reduces the concentration of the API in solution, but may not necessarily remove it from the environment. The extent (efficiency) of binding is dependent on the nature of the solid and thus will be location dependant.
Loss to air is almost never seen for most APIs, therefore the two main environments are water (major) and land (minor).
Persistence, Bioaccumulation & Toxicity (PBT)
Persistence, Bioaccumulation & Toxicity (PBT) are used to determine whether a substance poses concern for the environment. These properties can combine and lead to substances that are, for example, very persistent and very bioaccumulative (vPvB). As a result of the threat PBT/vPvB substances can pose, substances need to be screened for possible PBT properties under REACH (find out more about REACH – here). [3]
- Persistence (P) – the compound is recalcitrant and not biodegraded hence will persist in the environment.
- Bioaccumulation (B) – concentration of materials from water into organisms, usually in lipids/fats. This property is very difficult and expensive to measure in vivo and usually the octanol/water partition coefficient (Kow) is used as a surrogate indicator of bioaccumulation.
- Toxicity (T) – kills or is deleterious to microorganisms/animals. It is worth noting, however, that Endocrine Disruptor (ED) issues are more complicated: toxicity can depend on organism life stage, e.g. infancy vs adulthood. Additionally, toxicity may be indirect, making an organism more prone to infection or disease by e.g. triggering changes in DNA leading to mutation, or promoting the development of enzymes that inactivate antibiotics.[4]
Decomposition Routes
In the environment, there are three main decomposition routes for API molecules or their metabolites:
1) Chemical: typically, simple hydrolysis occurring between pH 5 and 8 – the pH will depend on the exact aquatic environment. Owing to the stability designed into most pharmaceutical molecules, uncatalysed chemical reactions in the environment may be limited to the hydrolysis of esters and possibly oxidation of highly reactive groups like mercaptans. Chemical transformations will not lead directly to mineralisation, but to a chemical species closely related to the original API which could be resistant to further chemical-only transformations. Most API molecules are resistant to oxidation by air, but may be oxidised in the presence of catalysts.
2) Enzymatic (or biotic degradation): molecules can be absorbed by microorganisms or higher life forms and metabolised via enzyme catalysis. Xenobiotic materials are metabolised to make them more polar to aid excretion, or to begin a breakdown process to utilise the molecule as a carbon/nitrogen source. These enzymatic transformations tend to follow human metabolism pathways – oxidation, reduction, hydrolysis and other less common transformations. API molecules may encounter enzyme classes common to humans but with different selectivities (such as P450s, a superfamily of haemoproteins that catalyse the metabolism of a large number of clinically important drugs), and classes of oxidative enzymes not normally utilized in human metabolism. As with humans, there can also be secondary metabolism – formation of phosphates, sulphates, and glucuronides. It should be noted that occasionally transformations in the environment can reverse the beneficial metabolic transformations in patients; one of the most problematic API molecules, EE2, is partly excreted as the soluble glucuronide which does not have estrogenic and endocrine disruption properties. This is converted back in STPs to the parent API which exhibits these undesirable properties, Figure 1.
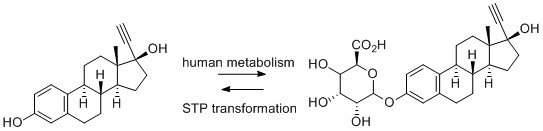
3) Photolytic decomposition/photochemical oxidation: direct photolytic decomposition (promotion to an excited state followed by a reaction) requires absorption of natural sunlight by the molecule (e.g. has an absorption maxima >290 nm). If a molecule has a UV/Vis maximum below 290 nm, it may still be decomposed by an indirect photochemical oxidation process, through reaction with high energy species like the ROO., OH. radicals and singlet oxygen, which are generated photochemically via dissolved sensitizers based on organic substances like humic acids. (More information on photodegradation and its impact on manufacturing, packaging, storage and testing of pharmaceutical products can be found in this article).
Most APIs are degraded in vivo via enzyme catalysis in microorganisms or in vitro by direct/indirect photochemical pathways.
"*" indicates required fields
- M. Bergheim, R. Gminski, B. Spangenberg, M. Dębiak, A. Bürkle, V. Mersch-Sundermann, K. Kümmerer and R. Gieré, Recalcitrant pharmaceuticals in the aquatic environment: a comparative screening study of their occurrence, formation of phototransformation products and their in vitro toxicity, Environmental Chemistry, 2014, 11, 431-444.
- X. – H. Wang and A. Yu- Chen Lin, Is the phototransformation of pharmaceuticals a natural purification process that decreases ecological and human health risks?, Environmental Pollution, 2014, 186, 203-215.
- European Medicines Agency (Last accessed: September 2022).
- E. Rahman Kabir, M. Sharfin Rahman and I. Rahman, A review on endocrine disruptors and their possible impacts on human health, Environmental Toxicology and Pharmacology, 2015, 40, 241-258.
- Examining the Life Cycle
- Drivers Towards Whole-process Thinking
- Challenges in Effecting Change
- LCA Examples
- Primary Manufacturing
- Secondary Manufacturing
- Packaging
- Pharmaceuticals in the Environment (PIE)
- Appendix: Carbon Footprinting Assumptions
- Examining the Life Cycle: Quiz
- Examining the Life Cycle: Summary and Further Reading
- The Fate of APIs
- Benign by Design
- Life Cycle Impacts: Summary and Further Reading